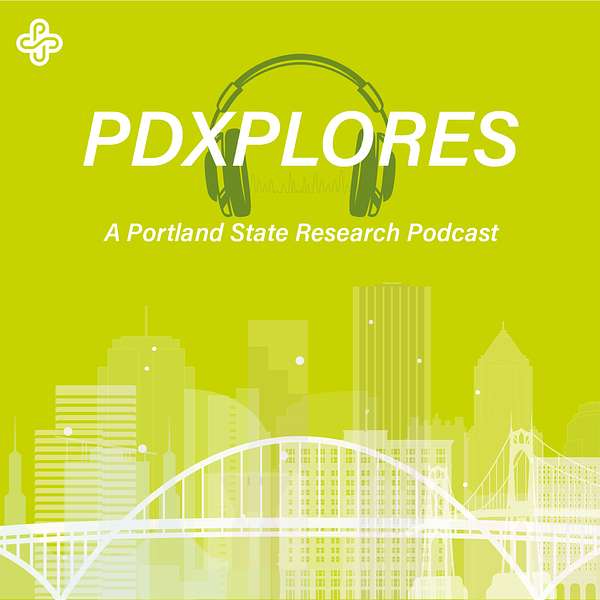
PDXPLORES
PDXPLORES
Exploring Extreme Viruses with Ken Stedman
In this episode of PDXPLORES, Ken Stedman, Professor of Biology at Portland State University, discusses his distinguished virology career. From discovering his love of extreme viruses at Max Planck Institute for Biochemistry in Munich, Germany, to heading the Advanced Molecular and Cell Biology Research Laboratory and the eXtreme Virus Lab, Steadman has investigated extreme virus structures, function, genetics, and evolution for more than 25 years. He is the co-founder of the research lab Center for Life in Extreme Environments as well as the Chief Scientific Officer of StoneStable, Inc. Adding to his scientific accomplishments and accolades, Professor Stedman is this year’s recipient of PSU’s Presidential Career Research Award.
Follow PSU research on Twitter: @psu_research and Instagram: @portlandstateresearch
Welcome to PDXPLORES, a Portland State Research podcast featuring scholarship innovations and discoveries, pushing the boundaries of knowledge practice and what is possible for the benefit of our communities and the world. My name is Ken Stedman. I'm a professor of biology and I'm also in the Center for Life in Extreme Environments. As a professor of biology, I teach a number of courses including a class I call Mutant Viruses from Hell, which is actually officially named the Advanced Molecular and Cell Biology Research Lab. I also teach molecular biology and virology. In our research in the Extreme Virus Lab, in the Center for Life and Extreme Environments at Portland State, we work on viruses and particularly viruses that are not the kind that make you sick. These are really weird and wonderful viruses from different parts of the world. Really extreme environments, as you might say. And in those we're really interested in what's the evolution, what are the structures? And also very interested in the persistence of these viruses. And that turned into a company which I started a couple of years ago, called Stone Stable, which is trying to make vaccines and gene therapies better. I first got interested in gene regulation when I first started learning about biology. My undergraduate degree is in chemical engineering, so something completely different than what I'm doing now. I took one biology course as an undergrad. I'm a biology professor, but in that class I heard things like, well, in any given cell in our body, the genes in our nose are exactly the same as the genes in our stomach or our ears or in our brain. But how those genes get turned on or turned off really determines what makes a nose a nose, a brain a brain or a stomach a stomach. So I thought, this is really fascinating and I'd love to know a bit more about this. So when I started graduate school, this kind of gene regulation, something I'm really interested in, but studying it in humans is really, really, really complicated. And given my background as a chemical engineer and very much a reductionist said, let's look at a system we're really gonna be able to understand at a molecular level how all of these things work. So I did my PhD work with Professor Sydney Kustu at UC Berkeley; probably the smartest person I'm ever going to meet. And in her group, we were working on gene regulation in bacteria. Literally how bacteria responded to various different sources of nitrogen. Do they like nitrate, nitrates, et cetera. But it turned out that in that system, and something that Dr. Kustu had discovered, was that the way these particular genes in bacteria were regulated was actually really similar to the way, at a much simpler level, that human genes were being regulated and turned on and turned off. So that got me, you know, really excited about this system. And then after I got my PhD and exercise, I just about as a finishing, I was teaching a microbiology course. I was the teaching assistant for the microbiology course that Dr. Kustu was the lecturer for. And I heard about these things called archea and I was like, whoa, these are bizarre. These are organisms that can thrive in boiling acid environments. And everything I'd learned about biology said, boiling acid's not terribly good for biology. So that was like, okay, these things are really cool. And then, because I've been working on gene regulation, the question was, well, what happens in gene regulation in these organisms that can live in boiling acid? And it turns out that at the very basic level, the way that those organisms turn their genes on and off, even though they look like bacteria, is actually more like you and me and human gene regulations. So I said, okay, after I'm done with our PhD, this sounds like a really interesting area to get into; organisms in crazy environments; wow, that's cool. And then how their genes are being regulated that could then potentially form some really interesting basal knowledge to understand how genes are being turned on and turned off in humans. So I looked around and tried to find who was working on these things, and it turns out there were four labs in the whole world that were working on transcription regulation in archaea at that point. So I went and visited all four of those labs. Two in Germany, one in England, and actually the one in Ohio actually didn't go and visit, but I visited the ones in England and the two in Germany. And then the one I visited in Germany was run by a fellow by the name of Professor Wolfram Zillig. And he was the one actually had discovered that in archaea, the gene regulation was more similar to what was happening in us than it was to other kinds of bacterial systems like I'd been working on for my PhD. And Wolfram Zillig is an amazing character. I could spend an hour talking about him. But after I visited in his group at the Max Planck Institute for Biochemistry just outside of Munich, I said, I need to work with this guy. This is just too fascinating and too interesting in terms of something that I really wanted to study. So I went to work with Wolfram Zillig, worked there for three years, looking at trying to understand gene regulation in archaea. The main reason to work with viruses, and particularly these extreme viruses, the viruses infecting archaea, was that to really understand gene regulation, there are kind of two different ways of doing that. There's something called biochemistry, which is where you purify all the individual proteins and all the things in the DNA, put them together in a test tube and see how they work. And then there's a method called genetics, which is, I always like to say what geneticists do is they break stuff and then they try and figure out what they broke, and that tells you what's going on actually inside the organism. One of the things that I actually knew about what was happening in gene regulation in archaea was that. The biochemistry is actually pretty good. People have been able to purify proteins, put them together. This is actually how they showed originally that they were more similar gene regulation to what was going on in humans. But what they didn't have was a good genetic system. And so when I mean a genetic system, that's a way of moving DNA into and sometimes out of cells. What turns out viruses have evolved for billions of years to do exactly that. They move genetic information in and out of cells so we, the scientists, can take advantage of the fact that a lot of these viruses have figured out how to move DNA around and try and use those as tools to understand the organisms that they're infecting. And this is something people have been done for literally decades now. The very first discovery that DNA was the genetic material was done in the study of viruses that infected bacteria. So it turns out that almost nothing was known about viruses that infected archaea at the time, other than actually a lot of work that had been done in the lab of Wolfram Zillig, which is where I was studying gene regulation. So that's how I started studying the viruses, was to use them as genetic tools. But in order to use them as genetic tool, you need to understand how the virus works. So that really got us to think much more about, oh, how is the virus working? We've still done some experiments looking at using them as genetic tools to study gene regulation, but the viruses in and of themselves are so fascinating, so interesting that our researchers really turned much more in the direction of,"Oh, how are those viruses working?" rather than looking at the gene regulation? So where I first discovered my love of extreme viruses was very much in working in Wolfram Zillig's lab as a post-doc at The Max Planck Institute for Biochemistry. But part of that was also, while we were there, we went on a field trip to place just outside of Naples, Italy called Pirelli close to Pozzuoli solfatara. The original name of some of the organisms we work with is sulfolobus sulfertagus, from the solfatara. There, we actually collected samples from some of these boiling acid springs. And so that to me was really an epiphany and said, "Hey, this is something that I could really sort of feel that I was working with," as opposed to a lot of the stuff I'd done during my PhD work, which was little dots on pieces of paper that I really didn't feel that connected to. And one of the things that this has led to is now our research group actually tries as often as possible, usually about once a year, to go to some of the local hot spring environments. And local for us is Northern California; only a nine to 10 hour drive away to Lassen Volcanic National Park, just to be able to observe and collect samples from some of these environments that these things live in outside of the lab. The main thing about extreme viruses is the thing I mentioned right at the beginning about archaea, how can something survive in boiling acid? That still blows my mind in terms of how they can do that. So that's really the thing which has gotten us interested. You know, what is it about their structure? What is it about their evolution? How can they then really survive under these really extreme conditions? I did have this, I called an epiphany, when I did go out into the field and for the first time, saw these bubbling hot springs and said, "Wow, I'm working on something like real and legitimate that I can actually think about and really visualize a real environment where the things are that we're working on are in." So that was one thing when we made some pretty fundamental discoveries. Couple of times actually. One was when I discovered a way to make a whole bunch more of this virus in different cells. In this case we called it a shuttle vector so the virus could make more of itself in a bacterium, but also inside the archaea. And this process has allowed us to do huge amount of work, including in the Mutant Viruses from Hell Lab that I talked about a little bit earlier. If we didn't have that tool, we wouldn't have been able to do any of these things. So that was a huge breakthrough and I even thought to myself at the time, "This is really, really cool. This is really gonna be a breakthrough." Second time was when I know that I was probably the first person ever in the world to see a brand new virus in a hot spring sample that we collected at that point in Yellowstone National Park. And then the third time was actually more different because we actually found something that we at first didn't believe. And then as we started looking more and more into it, and particularly graduate student Jeff Demer, who was working in the group at the time, we convinced ourselves that this new gene that we'd found a new virus actually was something completely different and something that nobody had ever at least not reported on before. Later we actually found out people had seen it and ignored it because they didn't know what to do with it. So that was, uh, pretty exciting. So the qualities that fascinate me the most about these extreme viruses is really that they can survive under these really extreme conditions. High temperatures. The high temperatures are about 80 degrees Celsius. It's about 170 degrees Fahrenheit, and a pH of two or three, which is around lemon juice, not quite as much as stomach acid. So that still blows me away that there's something about biology that can survive under those conditions. So that's probably the most interesting thing, I think, in terms of being inspired to think about doing the study of these things. The Advanced Molecular and Cell Biology Research Lab, again, a course at Portland State University, which we also call Mutant Viruses from Hell. This is something we call a course-based undergraduate research experience or CURE, C U R E. And one of the reasons we do this is that this is an opportunity for many more students to get involved in actual research than we can have in a particular research lab, such as the Extreme Virus Lab. So this advanced molecular and cell biology research lab, on day one, all the students get mutants of our favorite virus, which is a virus called SSV 1, Sulfolobus Spindle-Shaped Virus 1, the original virus that was discovered that infected these extremophilic archaea, one that I've been studying for over 25 years now, still a lot that we don't know about this virus. So we've made random mutants in the virus genome, and we don't know if these viruses work; they don't work; we don't know where the mutation is. And so this is what the students get. And over the next 10 weeks, they figure out what the mutation is. They can then make predictions in terms of what they think that mutation will do to the virus, and then can make experiments that will test those predictions. And the whole time, they're learning pretty classic techniques in microbiology recombinant DNA, virology, microbiology, and all of these are techniques that they can then apply hopefully for future jobs. The Mutant Viruses from Hell, these are literally these mutant forms of this Sulfolobus Spindle-Shape Virus, which kind of looks like a lemon actually, with a short tail at one end, these viruses grow optimally in boiling acid, so not quite boiling. It's only 80 degrees Celsius, so 170 some degrees Fahrenheit, and a pH of about two to three. Again, lemon juice, approximately. But a lot of people, when they find the environments that these things are from, will give them interesting names. And one of our favorite places to go and sample is a place called Bumpus Hell. The reason it's called Bumpus Hell is because a certain Mr. Bumpus in the late eighteen-hundreds was taking some tourists around some of these hot spring areas. He broke through the crust of the ground in these areas and ended up burning his legs so badly, both of 'em had to be amputated. So that is why it's called Bumps Hell and the Mutant Viruses from Hell. And in fact, when I give academic seminars, my title is almost always Viruses from Hell. I wish I could take credit for coming up with that. Actually, it was a reporter for US News and World Report who came up with this, um, viruses from hell. But, he's allowed me to use this, um, in terms of how we talk about the viruses that we're working on. So viruses from hell, there's literally these viruses you find in these extreme environments, and the mutants are the ones that we've actually made in the lab in order to try and understand how they work. In terms of which are the most, I wouldn't say mostly extreme viruses, but some of the mutants that we've found both through this teaching lab and also in our research lab, the Extreme Virus Lab at Portland State University, there are a couple of really unexpected ones. One of those, as I mentioned, these have a lemon shape. It turns out that that lemon shape is basically made up of two proteins that fit together to make this lemon structure, and we figured both those proteins are gonna be absolutely critical for how that virus works. Turns out we can get rid of one of them, and the virus is still okay. It doesn't look like a lemon anymore. It looks like a cigar. What's going on there? We really don't know. There's another one that was discovered actually in the teaching lab where it looks as if the virus wasn't working anymore when we made a mutation. But it turns out we've actually now figured out that the virus is still being made, it's just not making the cells as sick anymore. So it looks as if instead of it being a worse virus in terms of the infection, it's actually a better virus. And so we're trying to figure out exactly what that means. We got rid of a gene, which looks as if it's probably encoding something that makes the cell really sick. But it's still making a virus. That has really changed a lot about how I think about, usually think about viruses. You know, viruses make people sick, right? Viruses make microbes sick. But here it's a virus that is not making a microbes sick, but it's still making virus. So what's the interaction now between the cell and the virus when the virus is not making the cell sick anymore? So what's virus, silicification and purification? What this study is all about has to do with a lot of the interests we have in in virus evolution. And one of the big questions in virus evolution, and actually even in terms of where life came from even in the first place, is how old are viruses? We know that bacteria are probably four-billion years old, cuz if you look at the oldest rocks you can find on planet earth, in them, you find fossils that are pretty clearly bacterial in origin. But what about virus fossils? We know that all organisms today, bacteria, archaea, humans, you name it, they've all got viruses associated with them. Some of the theories about how viruses arose, and how life arose, postulate that you also have have to have had something like a virus, anyway, very, very early on in life, but there's nothing like these fossils in really, really old rocks. So we said, "Well, why has nobody discovered virus fossils?" There are two possibilities to that. One is there aren't any. Hard to prove a negative. The second one is that maybe there are a bunch of virus fossils or viruses in rocks, we just don't know how to detect them. So what we thought was, well, if there are viruses in rocks, we're gonna find a virus in a rock. The place you're going to find a virus in a rock is incredibly conveniently, places near where we've already find these viruses from Hell because, the virus is from Hell, these volcanic hot springs, what they are is superheated water that's been in contact with the earth and then comes up to the surface and cools down. The superheated water leeches out all the minerals in the rock that is going through. It gets to the surface. It's now a super saturated solution. Now it cools down and what happens is it starts to form rock. And if you've been to places like Yellowstone, some of these really amazing places, some of the hot springs you see really throughout the world, where you have these massive amounts of mineral deposition. Well, we know there are viruses there. We work on those viruses. So what's happening now between these hot springs, with the very high minerals and the viruses, which we know are there. So we decided instead of going to look at the hot springs, we still need to know what we're looking for first. So what we did is we took a really well-behaved virus, a big one, kinda looks a little bit like a spaceship, and put that into an artificial hot springing environment in the lab where we could control all of the conditions. And what we found was, after a couple of days under these very high mineral conditions, we had these beautiful viruses coated in silica. We use silica because it's really easy to use. The most common molecule probably on our planet; it's what rocks are made of. And so that gave a beautiful glass coating around the virus particle. And we could see this in the electron microscope. But after about three or four days, this beautiful glass coated virus became an unrecognizable blob, and there are lots of unrecognizable blobs in rocks that are filled with organic materials. So there probably are lots and lots and lots of virus fossils in rocks. But, this was interesting. Again, we, you know, kind of expected it and you know, it was a nice result: got a nice publication out of it. But then with the student who's working on this, Dr. Jim Laidler, who's actually a medical doctor before he came and did a PhD with our group, he then said, well, what happens to these viruses when they get this coating of silica on the outside? Not surprisingly, these viruses lose their infectivity. Again, they're getting coated in glass and viruses have to interact with the cell in order to be able to in infect. So they got coated in glass. Well that was this lunar lander like virus. It's a bacterial virus that normally would never see these really high mineral conditions. So then what we did is we took our viruses from Hell, these SSV's that are normally present in the high mineral conditions, and what he saw was they lost infectivity too, but nowhere near as fast as the ones that would never have seen silica. They presumably evolved to deal with these, these high silica concentrations. We were doing these experiments and looking at how fast they were losing their infectivity and comparing the different viruses to each other. And then as is so true with many PSU students, Dr. Jim Laidler had to go home to his kids halfway through an experiment. He came back the next day and found to all of our surprise that instead of losing more infectivity, if he then just diluted the sample, he saw that the infectivity actually came back. What we were able to show was that this silica treatment, basically beginning of fossilization, was actually a way to reversibly inactivate viruses. So you coat them, they lose their infectivity, but you can uncoat it really easily just by diluting the sample that has those things in it. The other thing that Jim found was that once those viruses are coated in silica, they're actually really resistant to environmental extremes. So it's a way of preserving viruses by putting them in a silica coat silicification that then you can use to stabilize those particular viruses. So that turned into a number of patents through Portland State University Innovation, Intellectual Property Office. Now a startup company, which we started a couple of years ago, and now we're in the process of trying to show that this is something that can be used to stabilize not viruses that are making you sick, but there are a lot of vaccines that are based on viruses, including the Johnson and Johnson Covid vaccine, the AstraZeneca Covid vaccine that are all based on viruses that now we can stabilize so that you don't have to keep them in an ultra-deep freezer the whole time. You don't have to use it or lose it like it was early on in the whole Covid vaccination campaign. You know, poor guys stuck on the highway. All their samples have thaw out, okay? They pull people over and just start giving him shots. So this process, we think, could potentially then allow much better distribution of vaccines for a lot lower cost, and particularly in places of the world where they don't have reliable, deep freeze, what they call cold chain conditions, in order to get the vaccines to people who really need them. Interestingly enough, this virus silicification process, the virus fossilization, also works on lots of other kinds of biologicals. So we got some funding from the Bill and Melinda Gates Foundation to look at stabilizing parasite eggs in stool samples. And the reason for that is that the stool samples, in places where you have large amounts of parasitic disease, are usually being produced not where they're being analyzed. So you have to get those stool samples somehow from, again, where they're being produced to, where they're being analyzed. And so stabilizing them is a, a really big deal. Mostly because the way that people detect that there's an infection of some kind of parasitic worm disease is by looking at the eggs. And those eggs have a very, very distinctive structure. But there's lots of other things in stool that would love to get rid of those eggs, and so treating them in silica turns out to be a way that we can help with diagnosis. But again, this silica coating technique is incredibly generalizable, so we are looking at it now mostly through our company, Stone Stable. Why Stone Stable? Because it's silica. Silica are rocks, they're stones, and we're stabilizing them basically by making them into little tiny rocks. But it turns out that it's, again, it's not just viruses. It's not just parasitic worm eggs. It's also things like lipid nanoparticles, which are what's critical for the mRNA vaccines. So we're looking into all of these potential applications, again, particularly for stabilization purposes. Astrovirology , which we like to think is kind of a term that we made up. Um, when I get business cards printed, my new ones might actually say "Astrovirologists" on them. I think that would be kind of fun. But the idea here is that everything we know about Earth and life on Earth has viruses associated with them. And viruses are really clearly very important for evolution, for probably, actually maybe even inventing DNA in terms of a potential previous, what people like to call, an RNA world. So that's true on earth, but what about on other planets? If there's life on other planets? And this is something that we're very interested in, certainly from a NASA point of view. Are there viruses there? And this is the best kind of question because, it's really interesting if there are none there, and really interesting, if there are some there. If we were able to discover viruses on a sample from another planet, maybe even a Martian meteorite for that matter, then I think it's very clear evidence that there's some kind of cellular life there because all viruses have to infect a cell in order to make more virus. So what we've been thinking about is, "Okay, well how do you go about detecting viruses?" And this to some extent was the impetus for thinking about virus fossils. And so our original virus silicification projects were funded by NASA to look into, could be to take virus fossils on Earth, maybe could also to take virus fossils on Mars or on Enceladus. Something like that. That's one thing that we're thinking about from an astrovirology point of view. The second is that a lot of Earth's biogeochemistry - so cycling of carbon, cycling of iron, cycling of nitrogen that's almost all concentrated in the oceans - turns out is heavily influenced buy a lot of the viruses that are infecting the microbes that undergo a lot of these cycles, and my favorite example here is oxygen. How do you get oxygen? It's through photosynthesis. Photosynthesis from plants. Yes, there's a lot of that, but there's also a huge amount of photosynthesis from algae in the oceans. Well, turns out that. Some of the oxygen being produced by photosynthesis by algae in the oceans is due to virus infection, because some of these viruses bring genes with them that change the photosynthesis of the cells that they infect. And this is in order to make more viruses from the virus point of view, but is also, again, changing the amount of oxygen that we breathe, probably one in 20 breaths caused by a virus produced gene, which is kind of mind blowing. But again, maybe that's something which is happening on some other planets as well. And so people are thinking about how could you detect the presence of a virus? It might be able to detect the presence of a virus because different amount of oxygen is being produced, for instance, or different other kinds of nutrients which are being cycled. So that's another aspect of astrovirology. A third aspect of astrovirology is, humans to try and explore other places, the moon, Mars, et cetera, they're bringing a bunch of viruses with them. And so what happens to viruses in a space environment is also a really interesting and open question that we do not have a good handle on. So a lot of what astrovirology is is first appreciating the vast number of viruses that we have on Earth and thinking about them then in much more of a broader context, again, exobiology or an astrobiology kinds of context. So the idea here is really trying to integrate viruses and the study of viruses into astrobiology in general. We just wrote an article, which should be coming out actually relatively soon, to the International Journal of Astrobiology, where we go through some of these different aspects of where people should be thinking about viruses in an astrobiology context. The reason, again, is based on what we know about Earth. So these viruses clearly change biogeochemical cycles. But just interactions of viruses with their hosts are probably important for evolution of life as we know it. And some people even theorize, evolution of us. So we might not have grown as big or had as complicated genomes as we do without the presence of viruses. So finding viruses, or maybe actually even more importantly, not finding viruses in some other places, but still finding life would be really interesting to be able to compare what's happening in the presence and the absence of viruses. The other thing to think about is just flipping the whole question on its head. If there were an alien intelligence that came down and collected a sample from Earth and was looking in that to see if it could find signs of life, it would almost definitely sample ocean because most of our planet is covered in ocean and it wouldn't be able to collect a very large sample unless they've figured out anti-gravity, but we'll assume they haven't. They're gonna get a really should be small sample. And so that relatively small sample of seawater has millions to tens of millions of virus particles in it. They may have some bacteria, some archaea, definitely no whales or scuba divers. So, um, at least an alien intelligence sampling from Earth would make the conclusion is, we're a planet of viruses. So what about collecting in other samples? From other places, other worlds. Most of the funding from this research has come from NASA so far, but one of the reasons that we wrote an article about astrovirology and also put in what's called the decal survey, is to try and get more funding agencies interested in funding some of this work because it has been actually quite challenging to get funding for it. So the major impediment to this research, as always, is resources. So certainly funding is a big part of that, but also just getting people interested and involved. The more younger researchers we can get interested in, involved in studying some of these things, I think the more interest is going to be, and then again, more resources that will come. Being awarded the Presidential Career Research Award from Portland State University is really a validation of not so much what I have done, but much more about what the students and researchers have done in our group over the 20 plus years that I've been here at Portland State University. And it really makes me feel good that this is something that the university values. Viruses never ceased to amaze me, just because they can thrive in so many different conditions. They use so many different kinds of genomes. There's so many of them. And a lot of the things that we do, yeah, we get some experimental results and we don't understand what they are, but that doesn't matter because the viruses have figured these things out. So how is it they can survive under these really high temperature, low pH conditions, et cetera? And how are they evolving? How old are they? And one of the things that we found a couple of years ago now, we were looking in our favorite hunting ground in Lassen Volcanic National Park, a place called Boiling Springs Lake. Boiling Springs Lake, I like to call the largest hot spring in the world that almost nobody has ever heard of. It's a lake. Probably a hundred meters by a couple of hundred meters right off the Pacific Crest Trail. The low temperature of the lake year round is about 50 degrees celsius, so 120 odd degrees fahrenheit; far too hot to get into, and the pH is about two again year round. The high temperature in the lake is about 96 degrees celsius, around 200 degrees C. That's boiling at the 6,000 feet elevation that it's at. So this is perfect conditions for our viruses from Hell, right? So we went and collected there, found absolutely nothing. Now we now know, we think we know anyway, why this was the case. Viruses are all dependent on hosts, and so if you don't have the right host, you're not gonna find a virus that. It can infect and our colleagues at Humboldt State University have found appropriate hosts and so we could follow up on that. But right about at that time, this was in the late-2000's-ish , we then had an opportunity to do something called metagenome sequence. Now metagenome, think about genome sequences. That's sequencing all of our DNA, but a metagenome is sequencing a whole environment's DNA. So we said, well, let's do a metagenome of boiling Springs Lake and see what's there, but specifically for the viruses that are there. And we found some in all of those, it was about 400,000 sequences, some sequences that looked like our regular mutant viruses from Hell. So we know they're there, just we don't know the right exact way to look for them. But in the process of looking at all of these 400,000 sequences, graduate student, Jeff Demer noticed that there were some sequences in there that were really weird. Weird in the way that those sequences didn't look like any of our normal mutant viruses from hell, but they also looked more like plant viruses. And no plants can survive under those kinds of conditions. But even more weird was that those plant viruses are what are called RNA viruses, not DNA viruses. And these are generally two completely different kinds of viruses. You've got RNA viruses like Sars COV-2 , you've got DNA viruses like Mpox and vaccinia, and some of the microbial viruses. All of the other viruses we've been working with are all DNA viruses, and we'd sequence DNA from this metagenome, but we're finding sequences that look like RNA viruses. Very, very strange. At first, I didn't believe it. I told Jeff to go back and check his data. He went back and checked his data. We looked at it together and it turns out there are virus genomes. So what makes up a virus found in Boiling Springs Lake that about half of them look like an RNA virus and half of them look like a DNA virus. Very, very strange. No one had ever reported this before. Turns out people had seen it, but they'd ignored it in the past cause they didn't know how to understand it. So we then have been continuing to try and understand how this process could have occurred. How did DNA viruses and RNA viruses interact with each other? So, you know, Mpox plus Covid, you know, talk about really nasty kind of potentially emerging virus, but, these are not viruses that make any of us sick. So understanding this process, trying to figure out how these otherwise completely different kinds of viruses could be interacting with each other. And so this is a project we call the Crucivirus Project, because it feels like a crossing over between DNA viruses, RNA viruses, and we've been making a lot of progress. We still have a long way to go. Turns out we actually found some of these cruciviruses in the soil on the PSU campus, so it's a lot easier for us to get to than driving 10 hours down to Lasson Volcanic National Park. So it turns out these viruses are really pretty ubiquitous. You find 'em all over the place, but at very, very low concentrations. So they're challenging to work with, but we've got some neat ideas on how we think we're gonna be able to figure some of these things out. My name is Ken Stedman in the Extreme Virus Lab at Portland State University. We study viruses structure to figure out how they can survive under really extreme conditions of high temperature and low pH. We study virus evolution to understand the emergence of potential new viruses. And through studying these viruses, we discovered a technique that may allow vaccines to get to the people in the world who really need them. Thank you for listening to PDXPLORES. If you liked what you heard on this episode, please rate and follow the show anywhere you get your podcasts.